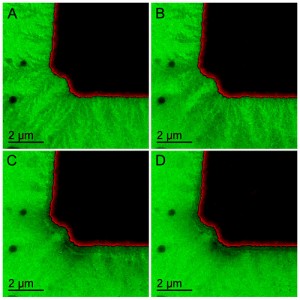
A new in situ transmission electron microscopy technique enabled ORNL researchers to image the snowflake-like growth of the solid electrolyte interphase from a working battery electrode. (Photo courtesy ORNL)
Using a new microscopy method, researchers at Oak Ridge National Laboratory can image and measure electrochemical processes in batteries in real time and at nanoscale resolution.
Scientists at ORNL used a miniature electrochemical liquid cell that is placed in a transmission electron microscope to study an enigmatic phenomenon in lithium-ion batteries called the solid electrolyte interphase, or SEI, as described in a study published in Chemical Communications.
The SEI is a nanometer-scale film that forms on a battery’s negative electrode due to electrolyte decomposition. Scientists agree that the SEI’s formation and stability play key roles in controlling battery functionality. But after three decades of research in the battery field, details of the SEI’s dynamics, structure, and chemistry during electrochemical cycling are still debated, stemming from inherent difficulties in studying battery electrode materials in their native liquid environment.
“We’ve used this novel in situ method to understand the dynamics of how this layer forms and evolves during battery operation,” said Raymond Unocic, ORNL research and development staff scientist.
Battery researchers typically study the structure and chemistry of the SEI through “post-mortem” methods, in which a cycled battery is disassembled, dried, and then analyzed through a number of characterization methods.
“This is problematic because of the air and moisture sensitivity of the SEI, and the ease by which environmental exposure can modify its structure and chemistry.” Unocic said.
The ORNL researchers formed a miniature electrochemical cell by enclosing battery electrolyte between two silicon microchip devices that contain microfabricated electrodes and silicon nitride viewing membranes. The transparent “windows” seal the highly volatile battery electrolyte from the microscope’s vacuum environment and allow the electron beam to pass through the liquid, which facilitates imaging of the electrochemical reaction products as they form.
To reproduce a battery charging cycle, the researchers applied a potential at the working electrode and monitored the resulting changes in current. The most striking result, said the researchers, was capturing an unprecedented view of SEI evolution during potential cycling. The technique is able to image the formation of tiny crystalline particles only one billionth of a meter in size.
“As we start to sweep the potential, we didn’t initially observe anything,” said lead author Robert Sacci, a postdoctoral research fellow with ORNL’s FIRST Energy Frontier Research Center. “Then we started seeing shadows—presumably polymeric SEI—forming into a dendritic pattern. It looks like a snowflake forming from the electrode.” Watch a video of the process at http://www.youtube.com/watch?v=OHrlFNB-Q9Y.
The researchers plan to build on this initial proof-of-principle study to better understand the factors behind the SEI’s formation, which could ultimately help improve battery performance, capacity, and safety at the device level.
“Tailoring the SEI’s structure and chemistry to maximize battery capabilities appears to be a delicate balancing act,” Unocic said. “When you cycle a real battery, the interphase structure can form, break, and reform again, depending on how thick the layer grows, so we need to look at improving its structural stability. But at the same time, we have to think about making the interphase more efficient for lithium ion transport. This study brings us one step closer to understanding SEI formation and growth.”
Next steps for the researchers include applying their technique to study different types of battery electrodes and electrolytes and other energy storage systems including fuel cells and supercapacitors.
Coauthors are ORNL’s Unocic, Robert Sacci, Nancy Dudney, and Karren More; and Pacific Northwest National Laboratory’s Lucas Parent, Ilke Arslan, and Nigel Browning. The study is published as “Direct Visualization of Initial SEI Morphology and Growth Kinetics During Lithium Deposition by in situ Electrochemical Transmission Electron Microscopy.”
The research was supported by the U.S. Department of Energy’s Office of Energy Efficiency and Renewable Energy, Vehicle Technologies Program, by the Fluid Interface Reactions Structures and Transport (FIRST) Center, an Energy Frontier Research Center funded by the Office of Basic Energy Sciences, and as part of a user proposal at ORNL’s Center for Nanophase Materials Sciences. Parts of the work were supported by the laboratory directed research and development program at Pacific Northwest National Laboratory and the Joint Center for Energy Storage Research, an Energy Innovation Hub funded by BES-DOE.
Part of this research was conducted at the Center for Nanophase Materials Sciences, which is sponsored at ORNL by the Scientific User Facilities Division in DOE’s Office of Basic Energy Sciences. CNMS is one of the five DOE Nanoscale Science Research Centers supported by the DOE Office of Science, premier national user facilities for interdisciplinary research at the nanoscale. Together the NSRCs comprise a suite of complementary facilities that provide researchers with state-of-the-art capabilities to fabricate, process, characterize and model nanoscale materials, and constitute the largest infrastructure investment of the National Nanotechnology Initiative. The NSRCs are located at DOE’s Argonne, Brookhaven, Lawrence Berkeley, Oak Ridge and Sandia and Los Alamos national laboratories. For more information about the DOE NSRCs, visit http://science.energy.gov/bes/suf/user-facilities/nanoscale-science-research-centers/.
Leave a Reply